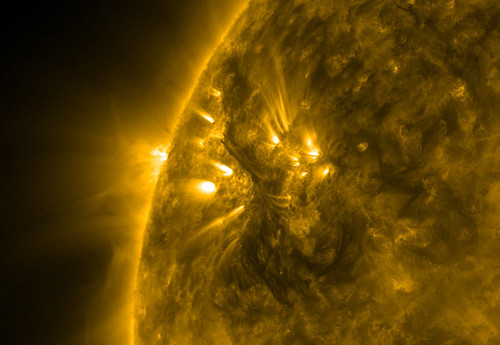
Image courtesy of NASA/Goddard.
The researchers found that decay rates changed by about 0.1% over the course of a recurring 33-day cycle. These measurements fit current theoretical models of the sun's inner rotation quite well. Interestingly enough, they also coincide with the occurrence of solar flares and correlate with the earth's yearly orbit around the sun. "Finding that the decay rates fluctuate in a pattern that matches known and theoretical solar frequencies is compelling evidence for a solar influence on decay rates," said Jere Jenkins of Purdue University, a lead engineer on the team.
The main suspect in this emerging investigation is the solar neutrino. Neutrinos are (nearly) massless, electrically neutral elementary particles that are only subject to the weak force; they can therefore travel extremely long distances without interacting with anything at all. Every second, the earth is pummeled with an innumerable amount of solar neutrinos. In fact, 65 billion neutrinos will pass though every square centimeter of your body in the time it takes you to read the word "radioactivity."

The first recorded evidence of a neutrino. A proton, muon, and pi-meson (right) leave diverging tracks that result from a single neutrino striking the chamber.
Image courtesy of Argonne National Laboratory.
So, if neutrinos don't interact with anything, how could they cause such changes in the decay of radioactive isotopes? Researchers don't really have an answer to that question. Jenkins' words say it best: "What we're suggesting is that something that can't interact with anything is changing something that can't be changed." However, if the team's data does turn out to be accurate, it could have broad implications. Scientists could use changing decay rates to predict and prevent the power outages and technological malfunctions that often accompany solar flares. Variable decay rates could also affect both archeological carbon dating and medical radiation treatments. Thanks to allegedly inert neutrinos, the sun's 11-year cycle might do more than just put on pretty light shows.